filmov
tv
Electron Transport Chain and Oxidative Phosphorylation
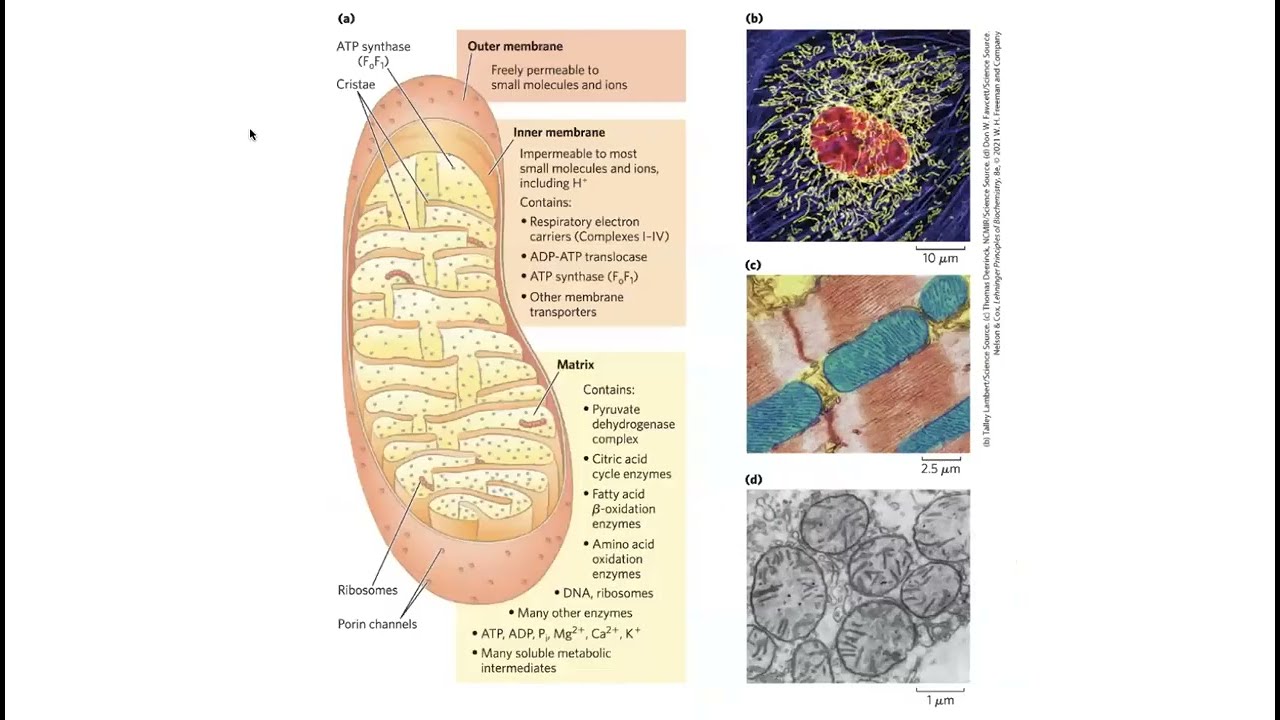
Показать описание
Similarly to how ATP is a “universal energy source”, NADH is a sort of “universal electron carrier” Nicotinamide Adenine Dinucleotide (NAD⁺) and it’s cousin NADP⁺ (just add phosphate) can accept electrons (get reduced) to become NADH and NADPH. It might look like the big difference between NAD⁺ and NADH is a hydrogen (H), but the real difference is “unspoken” – it’s the electrons! When NAD⁺ picks up that H, it picks it up as something called a HYDRIDE, which is an H with 2 electrons instead of its usual 1. Hydrogen atoms only have 1 proton & 1 electron & they often leave that electron behind when they go, so all they’re left with is a H⁺, so we often call H⁺ a proton but when H’s have 2 electrons we call it a hydride
NADH makes a great carrier because it wants electrons, but not desperately, so it’s willing to give and take. Some molecules want electrons more than others – and if something which doesn’t want or only kinda wants an electron (has a lower reduction potential) gives an electron to something that’s happier with it than it is (has a higher reduction potential) you get a net gain in happiness. And when you make molecules happier, they release energy (I like to think of it as them not having to fidget as much to get comfy so they can relax). So you can pass electrons from things with low reduction potential (like NADH) to things with high reduction potential (like O₂), and release little bits of energy as you do, which you can put to use - including to make ATP!
Electron-to-ATP conversion occurs in a process called OXIDATIVE PHOSPHORYLATION, which takes place in a secret club house in your cells called the mitochondrion (your cells actually have lots of mitochondria because of their important roles as cellular powerhouses). Unlike some other organelles (membrane-bound compartments inside your cells) like the endoplasmic reticulum (involved in protein processing), mitochondria have double membranes because they come from an ancient ancient ancient cell swallowing a bacteria and then adapting that bacteria to suit its needs, specializing in energy production through oxidative phosphorylation (oxphos).
OXIDATIVE PHOSPHORYLATION consists of an ELECTRON TRANSPORT CHAIN (ETC) and CHEMIOSMOSIS. The electron transport chain (ETC) occurs at the inner membrane (between the inner matrix & intermembrane space). Starting with NADH (or FADH₂), the original electron carrier transfers its electrons to another molecule that wants them more which passes them to another molecule that wants them even more . . . until they reach oxygen which wants them the most. Each of those pass-offs releases a little energy and that energy is used to pump protons out of the inner room of the mitochondria (mitochondrial matrix) into the outer room of the mitochondria (intermembrane space), creating a proton gradient where there are more protons in the intermembrane space than there are in the matrix.
Only one way back in is provided – through the ATP-making factory, ATP synthase, or as I like to call it, the ATP ATM. ATP synthase is seriously one of the most incredible machines ever made, able to harness the power of flowing protons similarly to how a hydrolytic dam harnesses the power of flowing water, in order to turn a molecular crank and turn ADP + Pi into ATP. It’s way more efficient than any man-made machine - and way way tinier. For each NADH that goes in, ~10 protons get pumped out, and it takes 4 protons coming in for 1 ATP to be made. 10/4= 2.5, so you get ~2 and a half ATP per NADH.
Pretty cool, eh? But you have to get the NADH in there (into the mitochondrial matrix) and spoiler alert - you can’t… At least not directly - but you can pass along the electrons its holding onto for you (which is the main thing you care about anyway). And then you can use those electrons to reduce more NAD⁺ inside the matrix so you end up with NADH in there without it ever crossing the inner mitochondrial matrix - sneaky, eh?
finished in comments
Комментарии