filmov
tv
MSG myth debunking and glutamate biochemistry
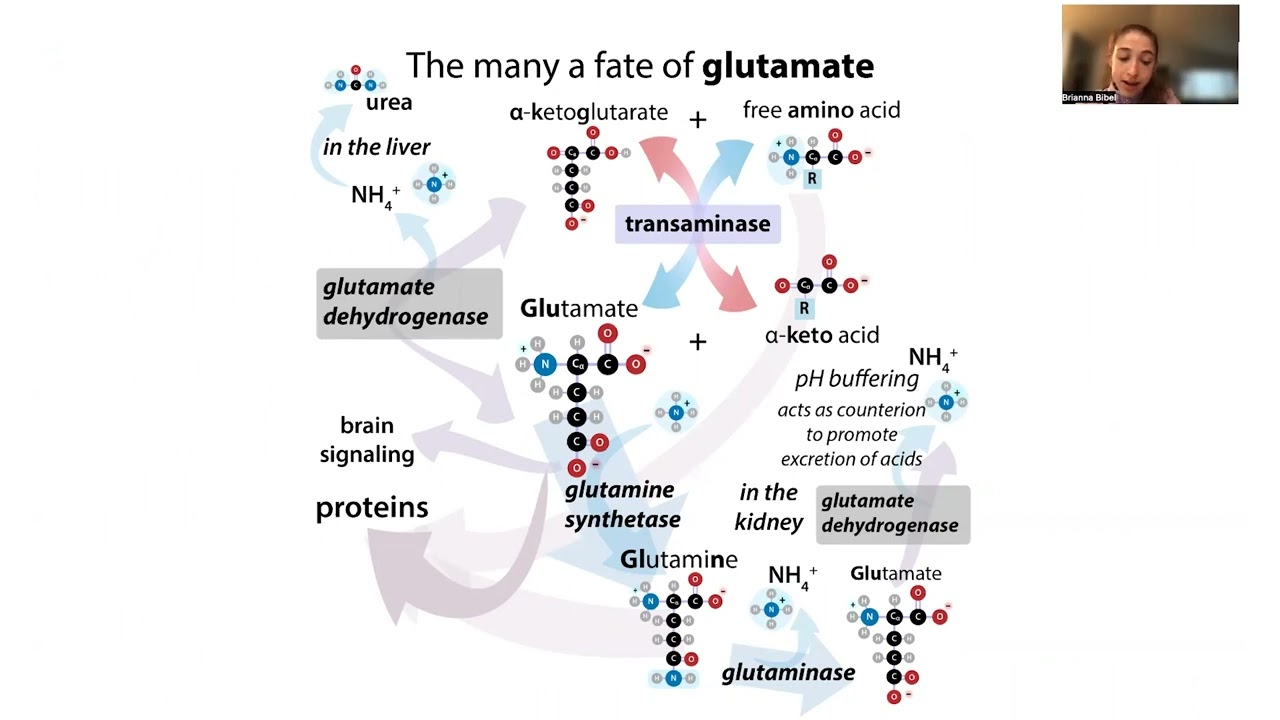
Показать описание
Glutamate, ionic “bonds,” salts, chemophobia, acidic amino acids, transamination, MSG myth, etc…. MSG? Fine by me! It’s just a salt of glutamate, so why does everyone gotta hate? From giving food its rich umami taste, to helping your cells safely remove nitrogenous waste, there’s a lot to love about this protein letter, so today I want to help you get to know it better! It’s more “popular” for its role as a brain messenger, but for those functions to brain experts I shall defer - instead, I hope you won’t become irate if I focus on some of the other (just as, if not more so) vital functions of glutamate (Glu, E)!
It’s Day 19 of #20DaysOfAminoAcids - the bumbling biochemist’s version of an advent calendar. Amino acids are the building blocks of proteins. There are 20 (common) genetically-specified ones, each with a generic backbone with to allow for linking up through peptide bonds to form chains (polypeptides) that fold up into functional proteins, as well as unique side chains (aka “R groups” that stick off like charms from a charm bracelet). Each day I’m going to bring you the story of one of these “charms” - what we know about it and how we know about it, where it comes from, where it goes, and outstanding questions nobody knows.
Those generic parts are attached to a central “alpha carbon” (Ca), which is also attached to one of 20 unique side chains (“R groups”) which have different properties (big, small, hydrophilic (water-loving), hydrophobic (water-avoided), etc.) & proteins have different combos of them, so the proteins have different properties. And we can get a better appreciation and understanding of proteins if we look at those letters. So, today let’s look at Glutamate (Glu, E)!
Glutamate is best known for its role a a neurotransmitter - a brain signaling molecule that nerve cells (neurons) use to communicate to other neurons. Want to send a message? - ship out some glutamate into the space between the cells (synapse) and that glutamate will bind to special receptors on the other cell, channels will open, and ions will rush in, leading to a change in charge that makes other channels open easier, sets off signaling cascades, etc. It’s cool stuff - but so many people find it cool that its role as an excitatory neurotransmitter tends to get all the spotlight. So today I want to tell you about some of the other aspects of glutamate that are just as - if not even more - important, yet are way further down the search results if you Google it.
Glutamate isn’t only important for neurons - it’s important for ALL our cells - really important - because it’s one of the key ways we can recycle amino acids while safely handling the nitrogen part. I’m going to tell you about this key role in “transdeamination” in a minute, but first I want to address something that might show up along with the brain stuff in your Google search - I’m talking about the myth that MSG added to food causes all sorts of symptoms like headaches, racing heart, tingling limbs, etc.
MSG stands for MonoSodium Glutamate and it’s just the sodium salt of glutamate. It gets added to food sometimes because glutamate is responsible for that rich “5th flavor,” umami. “Salt” is just another name we give to a neutral ionic compound - ions, by definition, are charged, thanks to their imbalance of protons & electrons (more below). So in order to get a salt you have to have a positive thing(s) (cation) and a negative thing (anion) - but you can mix and match. Stick a sodium ion (Na⁺) with a chloride ion (Cl⁻) and you get NaCl, or as you might know it better, table salt. If, instead of a chloride ion you stick the sodium with a glutamate anion, you get monosodium glutamate (MSG). Add in some disputed science and xenophobia and you get the myth that MSG causes a variety of strange symptoms.
I’m not just going to tell you it’s not true - it isn’t, but I want you to really understand what’s going on - especially since part of the reason the whole MSG myth has flourished is because of misunderstanding and chemophobia fueled by commercial opportunism (i.e. if it has a weird chemical name it must be dangerous so we’ll not use weird names on our labels…). Remember, dihydrogen monoxide is just a fancy name for water (and, for the lab nerds out there, DTT by any other name would still smell just as eggy) so don’t let companies profit off chemophobia!
finished in comments
It’s Day 19 of #20DaysOfAminoAcids - the bumbling biochemist’s version of an advent calendar. Amino acids are the building blocks of proteins. There are 20 (common) genetically-specified ones, each with a generic backbone with to allow for linking up through peptide bonds to form chains (polypeptides) that fold up into functional proteins, as well as unique side chains (aka “R groups” that stick off like charms from a charm bracelet). Each day I’m going to bring you the story of one of these “charms” - what we know about it and how we know about it, where it comes from, where it goes, and outstanding questions nobody knows.
Those generic parts are attached to a central “alpha carbon” (Ca), which is also attached to one of 20 unique side chains (“R groups”) which have different properties (big, small, hydrophilic (water-loving), hydrophobic (water-avoided), etc.) & proteins have different combos of them, so the proteins have different properties. And we can get a better appreciation and understanding of proteins if we look at those letters. So, today let’s look at Glutamate (Glu, E)!
Glutamate is best known for its role a a neurotransmitter - a brain signaling molecule that nerve cells (neurons) use to communicate to other neurons. Want to send a message? - ship out some glutamate into the space between the cells (synapse) and that glutamate will bind to special receptors on the other cell, channels will open, and ions will rush in, leading to a change in charge that makes other channels open easier, sets off signaling cascades, etc. It’s cool stuff - but so many people find it cool that its role as an excitatory neurotransmitter tends to get all the spotlight. So today I want to tell you about some of the other aspects of glutamate that are just as - if not even more - important, yet are way further down the search results if you Google it.
Glutamate isn’t only important for neurons - it’s important for ALL our cells - really important - because it’s one of the key ways we can recycle amino acids while safely handling the nitrogen part. I’m going to tell you about this key role in “transdeamination” in a minute, but first I want to address something that might show up along with the brain stuff in your Google search - I’m talking about the myth that MSG added to food causes all sorts of symptoms like headaches, racing heart, tingling limbs, etc.
MSG stands for MonoSodium Glutamate and it’s just the sodium salt of glutamate. It gets added to food sometimes because glutamate is responsible for that rich “5th flavor,” umami. “Salt” is just another name we give to a neutral ionic compound - ions, by definition, are charged, thanks to their imbalance of protons & electrons (more below). So in order to get a salt you have to have a positive thing(s) (cation) and a negative thing (anion) - but you can mix and match. Stick a sodium ion (Na⁺) with a chloride ion (Cl⁻) and you get NaCl, or as you might know it better, table salt. If, instead of a chloride ion you stick the sodium with a glutamate anion, you get monosodium glutamate (MSG). Add in some disputed science and xenophobia and you get the myth that MSG causes a variety of strange symptoms.
I’m not just going to tell you it’s not true - it isn’t, but I want you to really understand what’s going on - especially since part of the reason the whole MSG myth has flourished is because of misunderstanding and chemophobia fueled by commercial opportunism (i.e. if it has a weird chemical name it must be dangerous so we’ll not use weird names on our labels…). Remember, dihydrogen monoxide is just a fancy name for water (and, for the lab nerds out there, DTT by any other name would still smell just as eggy) so don’t let companies profit off chemophobia!
finished in comments
Комментарии