filmov
tv
Covalent Bonding Of Hydrogen, Oxygen & Nitrogen | Properties of Matter | Chemistry | FuseSchool
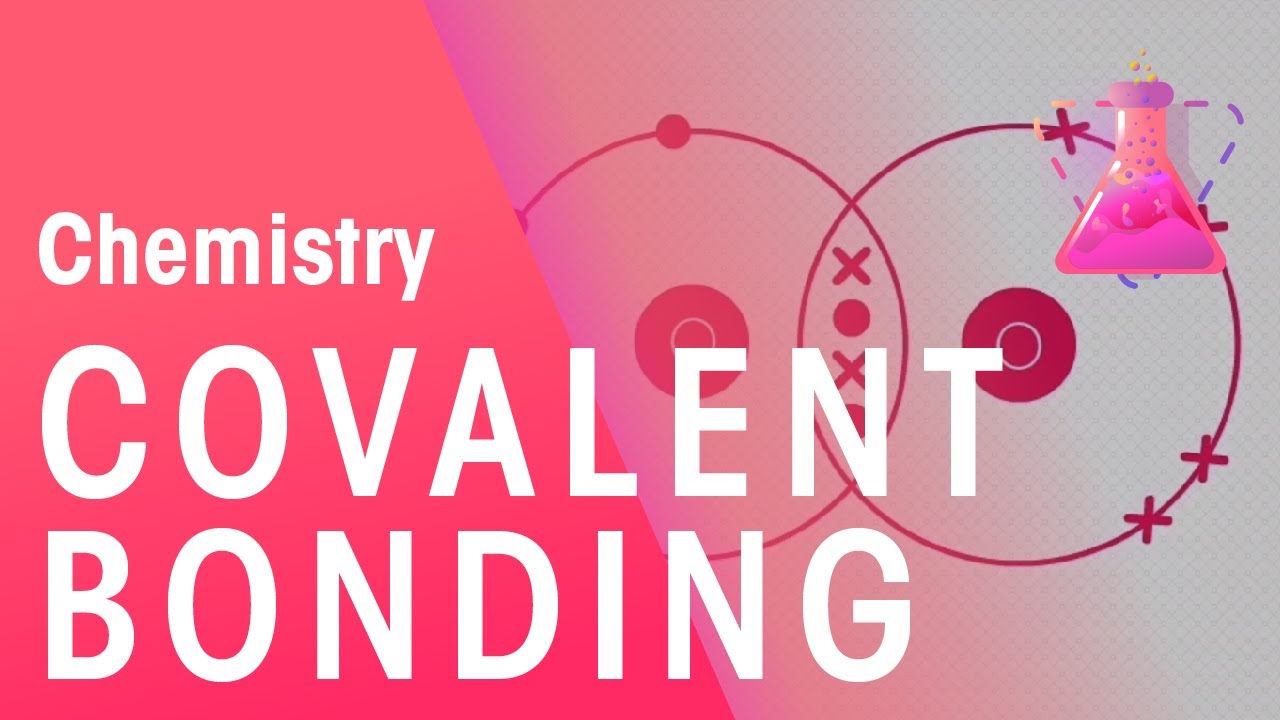
Показать описание
Learn the basics about the covalent bonding of hydrogen, oxygen and nitrogen as a part of the overall topic of properties of matter. The noble gas structure and covalent bonding is also discussed.
SUBSCRIBE to the Fuse School YouTube channel for many more educational videos. Our teachers and animators come together to make fun & easy-to-understand videos in Chemistry, Biology, Physics, Maths & ICT.
This video is part of 'Chemistry for All' - a Chemistry Education project by our Charity Fuse Foundation - the organisation behind The Fuse School. These videos can be used in a flipped classroom model or as a revision aid. Find our other Chemistry videos here:
SUBSCRIBE to the Fuse School YouTube channel for many more educational videos. Our teachers and animators come together to make fun & easy-to-understand videos in Chemistry, Biology, Physics, Maths & ICT.
This video is part of 'Chemistry for All' - a Chemistry Education project by our Charity Fuse Foundation - the organisation behind The Fuse School. These videos can be used in a flipped classroom model or as a revision aid. Find our other Chemistry videos here:
Covalent Bonding Of Hydrogen, Oxygen & Nitrogen | Properties of Matter | Chemistry | FuseSchool
Covalent Bonding Of Hydrogen, Oxygen & Nitrogen
covalent bonding H2O
Covalent Bonding of Hydrogen, Oxygen and Nitrogen
Covalent Bonding of Hydrogen, Oxygen & Nitrogen Chemistry for All The Fuse School
GCSE Chemistry - Covalent Bonding #16
Covalent Bonding of Hydrogen, Oxygen & Nitrogen
How atoms bond - George Zaidan and Charles Morton
JAMB CBT Chemistry 2024 Past Questions 26 - 50
Covalent Bonding of Hydrogen Oxygen & Nitrogen.
Chemical Bonding Introduction: Hydrogen Molecule, Covalent Bond & Noble Gases
Hydrogen Bonds - What Are Hydrogen Bonds - How Do Hydrogen Bonds Form
Covalent Bonding Of Hydrogen, Oxygen & Nitrogen | Properties of Matter | Chemistry | AddyESchool
Covalent Bonding - Dot and Cross Diagrams - p86
covalent double bond O2
How many covalent bonds can Hydrogen form?
Oxygen covalent bonding
formation of covalent bond in oxygen molecule
Covalent Bonding of Hydrogen, Oxygen & Nitrogen
Carbon and it's compounds (part 2)👉Covalent bonding #Hydrogen#Oxygen#Nitrogen
Single, Double, and Triple Covalent Bonds
Ionic and Covalent Bonds, Hydrogen Bonds, van der Waals - 4 types of Chemical Bonds in Biology
Ionic and Covalent Bonding - Chemistry
Bonds formed by Carbon | Don't Memorise
Комментарии