filmov
tv
Quantum Mechanics Explained in Ridiculously Simple Words
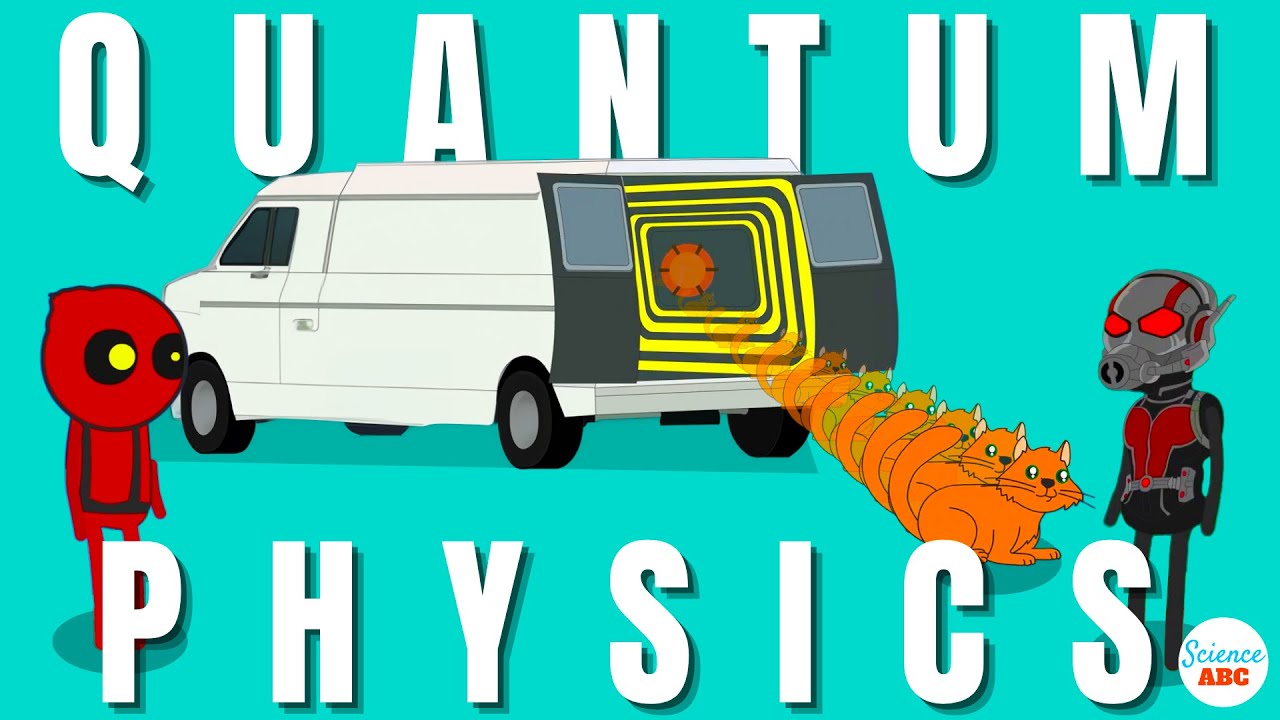
Показать описание
Quantum physics deals with the foundation of our world – the electrons in an atom, the protons inside the nucleus, the quarks that build those protons, and the photons that we perceive as light. These constitute everything that we are made of, both matter and energy. But how is it any different from regular physics? The 'regular' physics is the physics that deals with Newton’s laws of motion and its related mechanics. This is more formally called Classical Physics.
This is the physics of our day-to-day lives—the rolling of balls, the rotation of the earth, and the mechanics of engines. Quantum physics deals with particles that are incredibly small and hence, the laws of classical physics don’t apply in this world. In this video, we discuss the meaning of quantum physics and how is it going to change how we perceive the world around us.
#quantumphysics #quantummechanics #quantumworld
References:
SUBSCRIBE to get more such science videos!
Follow us on Twitter!
Follow us on Facebook!
Follow us on Instagram!
Follow us on LinkedIn!
Follow our Website!
This is the physics of our day-to-day lives—the rolling of balls, the rotation of the earth, and the mechanics of engines. Quantum physics deals with particles that are incredibly small and hence, the laws of classical physics don’t apply in this world. In this video, we discuss the meaning of quantum physics and how is it going to change how we perceive the world around us.
#quantumphysics #quantummechanics #quantumworld
References:
SUBSCRIBE to get more such science videos!
Follow us on Twitter!
Follow us on Facebook!
Follow us on Instagram!
Follow us on LinkedIn!
Follow our Website!
Комментарии