filmov
tv
The Incredible Story of Black Holes: Einstein, Schwarzschild, and Beyond
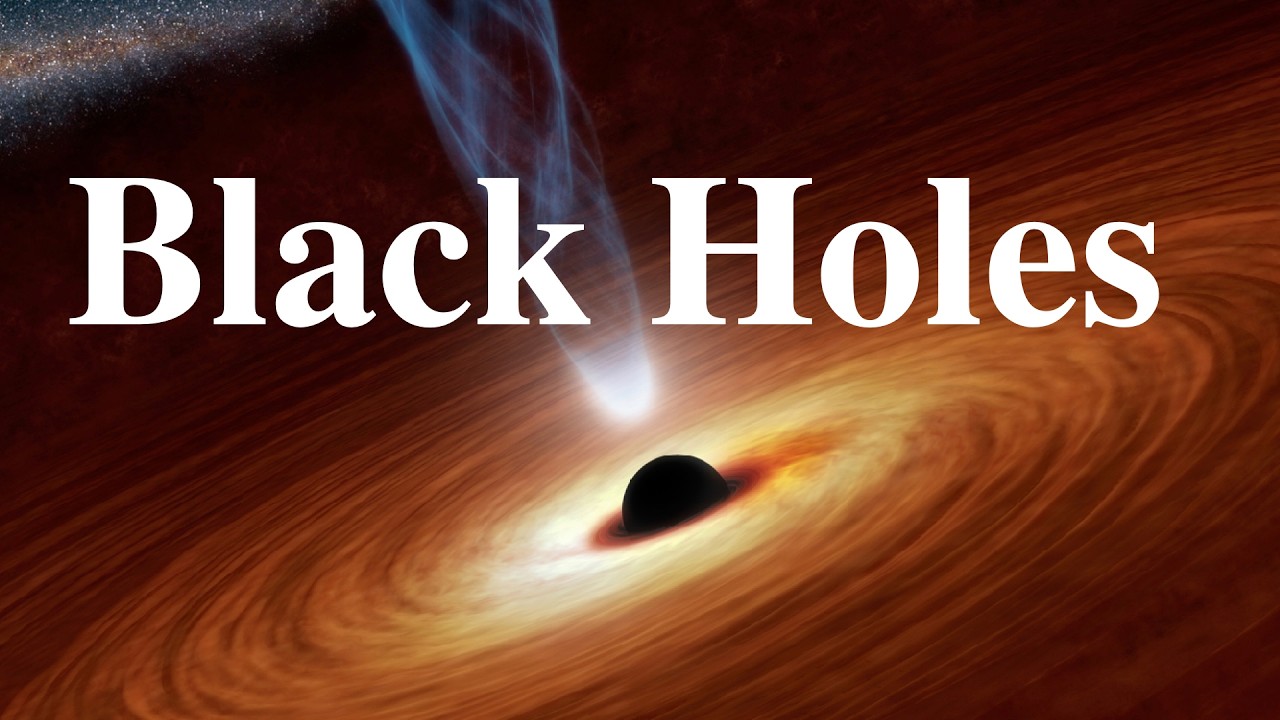
Показать описание
#BlackHoles #Astronomy #SpaceScience #CosmicMysteries #EinsteinTheory #Schwarzschild #GeneralRelativity #SpaceExploration #QuantumPhysics #Astrophysics
What you’re about to watch dives into the most massive, most compact, and most bizarre objects in the universe: black holes. I will guide you through the monumental triumph of gravity over matter, showcasing these cosmic endpoints and their enigmatic horizons into nothingness. It was Karl Schwarzschild who in 1915, while solving Einstein’s field equations of general relativity amidst World War I, created the first mathematical solution defining the spacetime around what we now refer to as black holes. In 1939, J.R. Oppenheimer and Hartland Snyder expanded on this by hypothesizing that when a massive star exhausts its nuclear fuel, it could collapse beyond neutron degeneracy pressure, forming an infinite singularity—a black hole. This collapse marks the fate of stars more massive than a few solar masses. Black holes are not merely empty spaces but regions of extremely warped spacetime where gravity reigns supreme, and escape is impossible. The Schwarzschild radius, or event horizon, defines this region where the escape speed exceeds the speed of light. For a black hole with the mass of our Sun, this radius is just three kilometers. Beyond the event horizon lies the singularity, a point of infinite density and zero volume. As you approach a black hole, the effects of gravitational redshift and time dilation become apparent. Light escaping from near the event horizon is stretched to longer wavelengths and loses energy. For an outside observer, time appears to slow near a black hole, leading to the phenomenon where an infalling object seems to freeze at the horizon. Understanding the anatomy of a black hole revolutionizes our perception of space and time. The intense gravitational fields warp spacetime so profoundly that not even light can escape once it’s past the event horizon. This immense curvature means that space is effectively “longer” near massive objects. We detect black holes indirectly by observing their gravitational effects on nearby stars and the X-rays emitted by infalling matter. The first widely accepted black hole, Cygnus X-1, discovered in 1964, showcases these traits. With a mass of about 14.8 solar masses, it emits X-rays as stellar material from a companion star falls into its event horizon. Recent advances have also allowed us to peek into the heart of galaxies, revealing that supermassive black holes, millions to billions of times the mass of the Sun, reside in their cores. For instance, the black hole at the center of our Milky Way, Sagittarius A*, weighs around 4 million solar masses and influences the motion of nearby stars. Movies like “Interstellar” have brought these cosmic giants into popular culture, depicting the awe-inspiring effects of their gravity on light and time. However, our understanding continues to evolve with the study of phenomena like Hawking radiation, where quantum mechanics suggests black holes can slowly evaporate over incredibly long timescales. Black holes come in various sizes: stellar-mass black holes stemming from the collapse of massive stars, intermediate-mass black holes, which remain a mystery, and supermassive black holes dominating the centers of galaxies. These enigmatic objects are pivotal in our study of the universe, from the smallest stellar black holes to the mammoth supermassive ones, each contributing to our understanding of gravity and spacetime. Join me as we delve deeper into the fascinating world of black holes, exploring their formation, properties, and the profound questions they raise about the fabric of the cosmos. See you soon!
This is part of my complete intro Astronomy class that I taught at Willam Paterson University and CUNY Hunter.
WOOPS LIST:
1) Just after 43:00, I misstated that all the light of the universe would fall into a black hole. Of course, that's not true. I should have said: "As you get closer to the singularity falling straight in looking up, you see all the light of the history of the universe that crosses the event horizon of the black hole you're in." Remember that a black hole is a very small target, however, light does pass through the event horizon. If the universe were to live to, say, 100 quadrillion years, then all the light that would ever enter the black hole for all that time would be seen by you all in a moment.
0:00 Introduction
2:11 Karl Schwarzschild
4:48 Earth compared to a White Dwarf
5:57 The size scale of a Neutron Star
10:41 Escape Speed if you shrank the Earth
12:54 Schwarzschild Radius
16:36 The Event Horizon
29:18 Gravitational Redshift
31:29 Gravitational Time Dilation
36:24 Spaghettification
37:40 Gravitational Lensing
40:50 Spiraling into a Black Hole
43:42 What's inside a black hole?
46:44 Measuring Spacetime Near Massive Objects
55:58 X-Ray Binaries
56:59 Black Hole Candidates
57:55 Observational Evidence for Black Holes
What you’re about to watch dives into the most massive, most compact, and most bizarre objects in the universe: black holes. I will guide you through the monumental triumph of gravity over matter, showcasing these cosmic endpoints and their enigmatic horizons into nothingness. It was Karl Schwarzschild who in 1915, while solving Einstein’s field equations of general relativity amidst World War I, created the first mathematical solution defining the spacetime around what we now refer to as black holes. In 1939, J.R. Oppenheimer and Hartland Snyder expanded on this by hypothesizing that when a massive star exhausts its nuclear fuel, it could collapse beyond neutron degeneracy pressure, forming an infinite singularity—a black hole. This collapse marks the fate of stars more massive than a few solar masses. Black holes are not merely empty spaces but regions of extremely warped spacetime where gravity reigns supreme, and escape is impossible. The Schwarzschild radius, or event horizon, defines this region where the escape speed exceeds the speed of light. For a black hole with the mass of our Sun, this radius is just three kilometers. Beyond the event horizon lies the singularity, a point of infinite density and zero volume. As you approach a black hole, the effects of gravitational redshift and time dilation become apparent. Light escaping from near the event horizon is stretched to longer wavelengths and loses energy. For an outside observer, time appears to slow near a black hole, leading to the phenomenon where an infalling object seems to freeze at the horizon. Understanding the anatomy of a black hole revolutionizes our perception of space and time. The intense gravitational fields warp spacetime so profoundly that not even light can escape once it’s past the event horizon. This immense curvature means that space is effectively “longer” near massive objects. We detect black holes indirectly by observing their gravitational effects on nearby stars and the X-rays emitted by infalling matter. The first widely accepted black hole, Cygnus X-1, discovered in 1964, showcases these traits. With a mass of about 14.8 solar masses, it emits X-rays as stellar material from a companion star falls into its event horizon. Recent advances have also allowed us to peek into the heart of galaxies, revealing that supermassive black holes, millions to billions of times the mass of the Sun, reside in their cores. For instance, the black hole at the center of our Milky Way, Sagittarius A*, weighs around 4 million solar masses and influences the motion of nearby stars. Movies like “Interstellar” have brought these cosmic giants into popular culture, depicting the awe-inspiring effects of their gravity on light and time. However, our understanding continues to evolve with the study of phenomena like Hawking radiation, where quantum mechanics suggests black holes can slowly evaporate over incredibly long timescales. Black holes come in various sizes: stellar-mass black holes stemming from the collapse of massive stars, intermediate-mass black holes, which remain a mystery, and supermassive black holes dominating the centers of galaxies. These enigmatic objects are pivotal in our study of the universe, from the smallest stellar black holes to the mammoth supermassive ones, each contributing to our understanding of gravity and spacetime. Join me as we delve deeper into the fascinating world of black holes, exploring their formation, properties, and the profound questions they raise about the fabric of the cosmos. See you soon!
This is part of my complete intro Astronomy class that I taught at Willam Paterson University and CUNY Hunter.
WOOPS LIST:
1) Just after 43:00, I misstated that all the light of the universe would fall into a black hole. Of course, that's not true. I should have said: "As you get closer to the singularity falling straight in looking up, you see all the light of the history of the universe that crosses the event horizon of the black hole you're in." Remember that a black hole is a very small target, however, light does pass through the event horizon. If the universe were to live to, say, 100 quadrillion years, then all the light that would ever enter the black hole for all that time would be seen by you all in a moment.
0:00 Introduction
2:11 Karl Schwarzschild
4:48 Earth compared to a White Dwarf
5:57 The size scale of a Neutron Star
10:41 Escape Speed if you shrank the Earth
12:54 Schwarzschild Radius
16:36 The Event Horizon
29:18 Gravitational Redshift
31:29 Gravitational Time Dilation
36:24 Spaghettification
37:40 Gravitational Lensing
40:50 Spiraling into a Black Hole
43:42 What's inside a black hole?
46:44 Measuring Spacetime Near Massive Objects
55:58 X-Ray Binaries
56:59 Black Hole Candidates
57:55 Observational Evidence for Black Holes
Комментарии